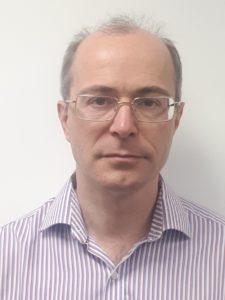
Stuart Baker is a professor of Movement Neuroscience at Newcastle University, Newcastle, England. He previously presented virtually on this topic at the UK Stroke Forum, 7–9 December.
We all take the ability to move for granted, and most of the time we do not notice how amazing our motor abilities are. But even something as simple as a sprained muscle can pull us up short, and remind us how much we rely on being able to move at will. For patients unfortunate enough to suffer more serious damage, such as after a stroke, the consequences are life changing. A busy and fulfilling schedule is swapped for a daily round of frustration.
In my group over the past 10 years, we have been studying the pathways that carry instructions to move from the brain down to the spinal cord, where they can activate the motoneurons which project to muscles. One pathway, the corticospinal tract, has been well studied. In primates like humans, it has developed extensively and is responsible for much of our motor control. It gives us fine dextrous abilities, needed for tasks such as playing the piano or threading a needle. But there are also other pathways, which arose earlier in evolution and we share with animals all the way down to frogs and fish – an example which we have especially worked on is the reticulospinal tract. These systems have generally taken the back seat in humans, although they still make a contribution. We typically think of them as controlling gross rather than fine movements – a punch, perhaps, rather than a caress – although our work has shown that, as so often in biology, it is all a matter of degrees, and actually all pathways play some role in all movements.
Understanding how different anatomical pathways contribute to movement might seem a niche academic activity, but it matters when it comes to thinking about recovery after damage. Typically a stroke damages the corticospinal tract, which is why the effects can be so devastating – the main system for controlling human movement has been knocked out. But once we realise that the evolutionarily older systems also make a contribution, even in healthy people, then it seems obvious that after damage they could be used to overcome the disability and mediate recovery. And actually, that is what happens: we have shown in experimental monkeys that after damage to the corticospinal tract, reticulospinal connections strengthen, partially restoring the lost inputs to the spinal cord and allowing some function to be regained. Yet, for reasons we do not completely understand, this happens in an imbalanced way. Connections to flexor muscles strengthen, but those to extensors do not. This leaves stroke survivors with a strong grasp, but often still unable to deploy the hand for useful tasks because they cannot open (extend) the fingers.
We wondered if we could intervene to rebalance the changes in the reticulospinal tract. In our monkey studies, we had serendipitously shown that reticulospinal cells can be activated strongly with loud click sounds – strange but true! We also know that there are strong inputs from sensory nerves in the limb, and that if two inputs to a neural system can be activated together with very close timing, this can strengthen output from the targeted system. So we developed a small ‘wearable device’, about the size of a smartphone, which would pair clicks with weak electrical shocks to a muscle. When healthy subjects wore this for a day, letting it click and stimulate in the background while they went about normal life, measures of reticulospinal function strengthened. We then carried out a trial of the device in stroke survivors, targeting connections to extensor muscles. After the volunteers wore the device for four weeks, there was a significant improvement in hand function.
Our work shows that clinical advances need to start with an understanding of the basic science; there is no substitute for a deep knowledge of the biology. Animal studies are of course key to this; we could never have made the advances we have without the work in monkeys, but this is no use in isolation; sooner or later, we have to test ideas out with human volunteers too. Finally, just showing benefit in a clinical trial is not enough; I want to see these approaches being used in all patients who could benefit. This is a major challenge at the moment, as the regulatory framework around Medical Devices imposes enormous and very costly barriers. The only way to go further is link with companies, but paradoxically, my wearable device turns out to be rather too simple to offer large profits for a company, and hence very hard to commercialise. Yet I remain hopeful. Neuroscience has made enormous advances in the last 50 years, and I think now is the moment we will start to see this translate into some really exciting benefits for patients.